The Energy Storage Solution
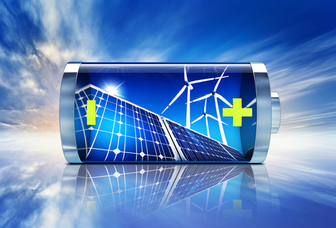
The ambitious targets regarding greenhouse gases (GHG) reduction recently fixed by governments are encouraging the development of renewable energy technologies around the world.
However, due to the inherent intermittent nature of renewable energy technologies (wind does not always blow, sun does not always shine, waves are not always in motion, rain an run-off does not always fill the hydraulic dams), challenges arise when trying to synchronise and balance supply and demand across the grid.
Energy storage provides a potential solution for this: it would enable to utilise the non-dispatchable “wrong-time” electricity generated from renewable energy sources at times when demand is greater than the addition of baseload generation plus the renewable contribution.
On top of the aforementioned aspects, storage offers other advantages such as (IMechE 2014):
However, due to the inherent intermittent nature of renewable energy technologies (wind does not always blow, sun does not always shine, waves are not always in motion, rain an run-off does not always fill the hydraulic dams), challenges arise when trying to synchronise and balance supply and demand across the grid.
Energy storage provides a potential solution for this: it would enable to utilise the non-dispatchable “wrong-time” electricity generated from renewable energy sources at times when demand is greater than the addition of baseload generation plus the renewable contribution.
On top of the aforementioned aspects, storage offers other advantages such as (IMechE 2014):
- Control of seasonal fluctuations (e.g. blackout risk in winter)
- Increase in ROI (Return On Investment) for renewable energy technologies
- Absorption of costs associated to the upgrade of the distribution system
- Enabling communities to be self-sufficient in managing their own energy
- Increase in resiliency and mitigation of impacts of extreme weather phenomena (recent examples of the potential effects of storms and floods can be found here and here)
- Citizen engagement towards a decarbonised economy
Storage technologies
Since electricity is an energy vector and not a form of primary energy, it cannot be stored. Hence, storage technologies require a preliminary conversion into a primary form of energy before accumulating it: mechanical, chemical or thermal.
A broad range of storage techniques have already reached either the commercial stage or the development phase. Their main characteristics are detailed below. Most mature and widely known is pumped storage. Consequently, it will be the only storage technology considered for this project (an additional comment about the possible usage of batteries as a form of storage is mentioned in the Conclusions section). Even though the other technologies were scoped out of this project, their main features are outlined below.
A broad range of storage techniques have already reached either the commercial stage or the development phase. Their main characteristics are detailed below. Most mature and widely known is pumped storage. Consequently, it will be the only storage technology considered for this project (an additional comment about the possible usage of batteries as a form of storage is mentioned in the Conclusions section). Even though the other technologies were scoped out of this project, their main features are outlined below.
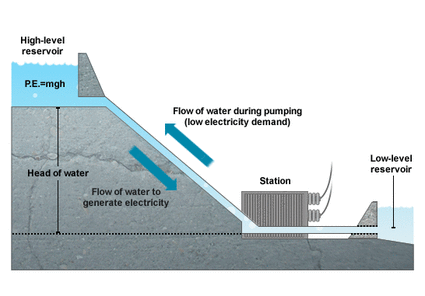
PUMPED HYDRO STORAGE
- Operation: in this case, energy is stored as gravitational energy of a fluid (water). Water is stored in upper and lower reservoirs, connected by tunnels. Water turns turbines when flowing from higher to lower reservoir as for conventional hydropower, and is then pumped back with off-peak power to recharge (EPR 2011).
- Existing technology: current power plants' rated power ranges from tens of megawatts to gigawatts, being Bath County (Virginia) in the United States the biggest one worldwide, with a generation capacity of 3,003 MW. Total installed capacity around the globe is about 104 GW, being EU, USA and Japan the main actors. Regarding projects under construction, China stands out in the lead.
- At a UK level, it needs to be mentioned there are 4 existing sites (Cruachan, Dinorwig, Foyers and Ffestiniog), providing 26.7 GWh of energy storage.

It should not be forgotten that pumped storage is a net user of power. However, its flexibility helps to cope with supply vs demand fluctuations and to resolve the intermittency associated with other renewable technologies.
In terms of cost of generation, the average CAPEX cost per kW of installed capacity ranges between $1,500-3,000/kW (£1000-2000). But it is in terms of generated energy where pumped hydro storage presents a very advantageous position, with a cost is $250-400/kWh (£175-275).
In terms of cost of generation, the average CAPEX cost per kW of installed capacity ranges between $1,500-3,000/kW (£1000-2000). But it is in terms of generated energy where pumped hydro storage presents a very advantageous position, with a cost is $250-400/kWh (£175-275).
- Future projects for the UK: new stations have already been planned (e.g. Coire Glas, with a rated power of 600 MW and a storage capacity of 30-40 GWh; Snowdonia - 100 MW - 0.6 GWh and Balmacaan - 300-600 MW, 30-4- GWh), as well as upgrades of existing sites (e.g. Cruachan, + 600 MW; Sloy +60 MW).
- Future developments: state of the art innovations include pumped hydro using sea water, as well as the utilisation of underground reservoirs.
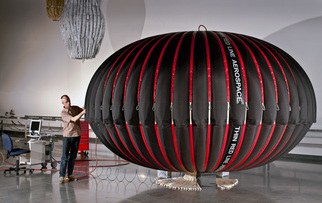
COMPRESSED AIR ENERGY STORAGE
- Operation: uses excess off-peak power to compress and store air. Air is later on used at times of high energy demand.
- Existing technology: 2 working plants globally – Huntorf in Germany and McIntosh in Alabama. Both use salt caverns as the storage vessel.
- Planned Plants: Gaelectric in Northern Ireland, which is in the advanced planning stage. ADELE in Germany is an adiabatic demonstration plant due to begin this year.
- Future potential:
- Gaelectric have another project for NW England in the early planning stage;
- Smaller scale: concept designs exist for subsea flexible pressure vessels.
SUPERCAPACITORS
- Operation: electrochemical capacitors which temporarily store electrical energy in an electric field.
- Features: very fast charge and discharge of load; broad temperature range of performance (-40 ⁰C to 65 ⁰C); semi-permanent life (hundreds of thousands of cycles); η = 95%; commercial volumetric energy density = 5 to 8 Wh/l; not proven in large scale storage.
- Future potential:
- Graphene supercapacitors: tens of thousands of charging cycles, store as much energy as lithium batteries and fast rate of charging and discharging. EV application or local distributed storage.
- Hong Kong testing supercapacitors on buses.
SUPERCONDUCTING MAGNETIC ENERGY STORAGE (SMES)
- Operation: electricity is stored by circulating a current in a superconducting coil, or inductor. As no conversion of energy to other forms is involved, round-trip efficiency can be very high.
- Features: used as Voltage and frequency stabilizers and load leveling; η = 95%; discharge greats amounts of power in short time; unlimited number of charges and discharges.
- Limitations and challenges: Requires cooling system to maintain operating temperature; expensive superconducting materials + mechanical supporting container; size limitations: 1 GWh -> loop of 100 miles -> huge land requirements.
- Existing technology:
- Tacoma-Washington substation, USA, 1976. Only case of SMES connected to the grid. 30 MJ unit;
- Currently small units used for power quality delivery.
BATTERIES
|
|
Potential increase in UK storage capacity
During the development of this project, we identified a significant potential increase in storage capacity for pumped hydro schemes in the UK, specially due to the favourable topography in Scotland. After a literature review and the corresponding research, it was found that storage capacity could raise by up to 400 GWh (MacKay 2008), or even 514 GWh (ESRU 2004).
However, we decided to maintain a more realistic, pragmatic and conservative approach and lower the expected increase down to additional 200 GWh, which seems to be achievable and was proved to be enough "to prevent the curtailment of renewable energy and reduce the use of CCGT’s as a load following mechanism" by Thomas Hoy in 2015.
In conclusion, we will consider a multiplication of storage capacity by a factor of around 9 from 2014 (26.7 GWh) to 2050 (26.7 + 200 = 226.7 GWh).
However, we decided to maintain a more realistic, pragmatic and conservative approach and lower the expected increase down to additional 200 GWh, which seems to be achievable and was proved to be enough "to prevent the curtailment of renewable energy and reduce the use of CCGT’s as a load following mechanism" by Thomas Hoy in 2015.
In conclusion, we will consider a multiplication of storage capacity by a factor of around 9 from 2014 (26.7 GWh) to 2050 (26.7 + 200 = 226.7 GWh).
References:
ESRU (2004), Scenario Analyses for Wind Penetration in Scotland; Renewable Energy Group Project, University of Strathclyde, Glasgow.
David J.C. MacKay (2008), Without the Hot Air; UIT Cambridge.
EPR (2011), The future role for energy storage in the UK (main report); The Energy Research Partnership.
IMechE (2014), ENERGY STORAGE: THE MISSING LINK IN THE UK'S ENERGY COMMITMENTS; Institution of Mechanical Engineers.
Thomas Hoy (2015), The Role of Additional Pumped Hydro Storage in a Low Carbon UK Grid; MSc Sustainable Engineering: Renewable Energy Systems and the Environment Individual Thesis, University of Strathclyde (Glasgow).