General principle
We have provided background information on heat pumps, standard water storage tanks and solar collectors which can be referred to for further information.Solar collectors capture heat from the sun during the day which used to heat water. The use of a storage tank (reasonably insulated - keep water warm for about 2 days) allows to collect and store all the solar energy that is available within the day until that tank reaches its normal storage temperature. Excess heat collected is either lost or may be collected in seasonal storage when applicable, but in our case we concluded that seasonal storage. The heat pump may be ASHP or GSHP, in colder climates a GSHP will be preferable. The functions of the heat pump include:
- Back up the solar collectors for domestic hot water
- Take care of space heating or cooling demand
The benefit of the heat pump is that it provides an efficient source of renewable heat when operating with a good COP value. The heat pump will consume a kWh of electricity for 3 to 4 kWh of heat (or cooling if reversible) it provides. The electricity consumed by the HP should either be renewable or be compensated for by renewable electricity (our project scope) injected into the grid at a later point.
Results
The key parameters to be considered here are: ◊ The space heating demand ◊ The space cooling demand ◊ The hot water demand ◊ The solar fraction
Solar collectors
Solar collectors will provide heat only at the time when there is solar power. There is no reason however why the time when solar heat is produced should correspond to when heat is needed for domestic hot water (or in some cases space heating).The reason to have a storage tank of a certain size is therefore to store solar heat produced so it can be used later, later here meaning short term storage based on daily requirements. The domestic hot water requirement is 240 litres per day based on 4 people in the dwelling. The hot water yearly demand is therefore 3560 kWh in Glasgow and 3051 KWh in Palermo. The water temperature in the tank vented cylinder is assumed to be low (45°C), this point) is discussed further later on. Solar collectors should be sized reasonably relative to the demand, in order to avoid over-sizing.
- Glasgow: A collector area of 2.8m2 provides 1675 kWh annually based upon monthly calculations, or a solar fraction of 47%. A more precise simulation would likely yield a slightly lower factor ~ 40%.
- Palermo: The solar heat yield is 2645 kWh, or a solar fraction of 87%.
A larger collector would provide more heat and increase the solar fraction but the additional heat will not just decrease the deficit, some of will be surplus which is of limited interest, so it makes sense to look at the deficit based upon area. This is shown on the graph below.
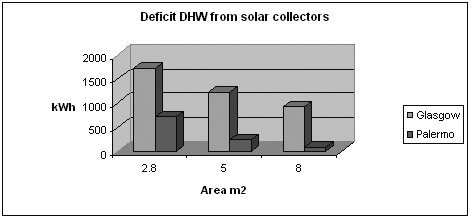
- Glasgow: If we go to 5m2 collector area we obtain a deficit 500 kWh smaller, which represents about 13% solar fraction. This improvement is largely insufficient to justify itself. The reason being that in Glasgow and alternative heat source is needed to make up for the solar deficit and the space heating, having a solar fraction of 60% will still require the same additional investment.
- Palermo: The benefit of using 5m2 collector area is even smaller, most additional heat produced will be surplus.
Another reason is regarding the physical integration of the system, increasing the solar collector size will impose additional constraints on this aspect. Solar collectors are therefore chosen with an area of about 2.8m2.
Heat pump
We now look at the benefits of using a heat pump for either dwelling. The complete hybrid system includes already ventilations systems (see the summary report and the analytical report) that allow reducing a major part of the original heating and cooling loads. Their contribution is included in the demand figures below. The figures below assume the solar fractions which are already reported in the previous section as. The HP in Glasgow covers DHW (non solar) and space heating, in Palermo it covers a small non-solar fraction of DHW and space cooling, it would be therefore a reversible HP, and most likely an ASHP.
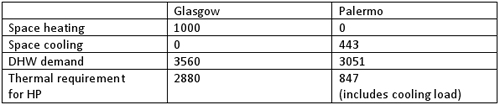
- Glasgow:
In Glasgow we simulate a standard model of GSHP ◊ Capacity is 5kW ◊ The COP is assumed to be 3 on average The results we obtained are: ◊ An electrical demand of 960 kWh for the HP ◊ A capacity factor of 6.6% ◊ The peak load is 2.3kW thermal output for the HP This is based on an optimised electrical demand which follows a regular pattern. In actual situation it is likely the peak load would sometime go above what has been simulated, so we believe 5kW is quite appropriate. The capacity factor is quite low due to the small demand in our low energy dwelling. This is expected.
If we remove the solar thermal system (simulated as a collector area = 0 m2) then the capacity factor goes up to 10.4% and the peak load is 2.5kW. We address this more in the financial analysis section which is presented below.
- Palermo:
In Palermo the heat pump requirement is 3.5 times smaller than in Glasgow. First, the DHW deficit heat is relatively small and could be provided electrically by a simple heating element in the tank. The cooling load is not high (443 kWh) and our assumptions regarding shading were conservative. In a practical situation, additional natural shading and external binds blocking all or almost direct sunlight would be in place to reduce the residual cooling load. We conclude therefore that there is no absolute requirement for a heap pump in Palermo, but it would provide some additional comfort level in summer.
Hybrid system using multiple heat sources - management of inputs/outputs using multiple temperatures
Let's look at the system we have in Glasgow. We have a requirement for DHW and for space heating.The HP can deliver heat to a low temperature system and use a standard under-floor heating for instance. The temperature output to such system would be about 28°C, the temperature of the water in the storage tank can be as low as 45°C. A standard control system monitors the temperature at the inlet of the under-floor circuit and regulates it by opening the valve to let warmer water in. The DHW is many conventional heating systems using a water storage tank which is kept at 60°C or more for bacterial control. This temperature from a practical point of view as well as an energy efficiency point of view is higher than it needs to be. It is possible to reduce this temperature to 45°C by using a UV system which takes care of bacteria. These systems are now fairly standard, for further information people may refer to the following references [1], [2], [3], [4], [5], [6]. Another possibility is to periodically heat up the water to above 60°C. The solar panels deliver heat to the storage tank when the temperature in the collectors is higher than the tank temperature by a few degrees. This is regulated by sensors which automatically circulate the water. We therefore have a centralised storage tank at 45°C with several heat sources input and outputs to space heating and DHW.
Financial analysis In the financial analysis we have to consider several parameters:
- The net investment cost, which depends on
◊ The equipment and installation cost ◊ Grants applicable ◊ The loan terms (interest rate and duration)
- The yearly maintenance cost (O&M) expressed as a percentage of the equipment cost
- The quantity of heat produced and the value of it
Yearly cost: The net investment cost and maintenance will be compiled into a yearly cost for the duration of the loan. The duration of the loan is set at what would be a reasonable lifetime for the equipment.Revenue obtained assuming all electricity produced is sold at a given value. The value of the electricity produced will depend on the renewable heat incentives. These are for the UK: GSHP: · 7 p/kWh · 18 p/kWh Net revenue: this is the revenue minus the yearly cost. We compare with a standard heating system. The cost of standard equipment is much lower than that of HP and solar collectors. As solar collectors cannot fulfil the demand on their own and the priority is to have a low-carbon system, we did not make a financial analysis of solar thermal on its own. We instead look at the HP and at the hybrid combination.
GSHP - Glasgow |